Suzie Sheehy is a physicist and author who divides her time between research groups at the University of Oxford and University of Melbourne.
Below, Suzie shares 5 key insights from her new book, The Matter of Everything: How Curiosity, Physics, and Improbable Experiments Changed the World. Listen to the audio version—read by Suzie herself—in the Next Big Idea App.
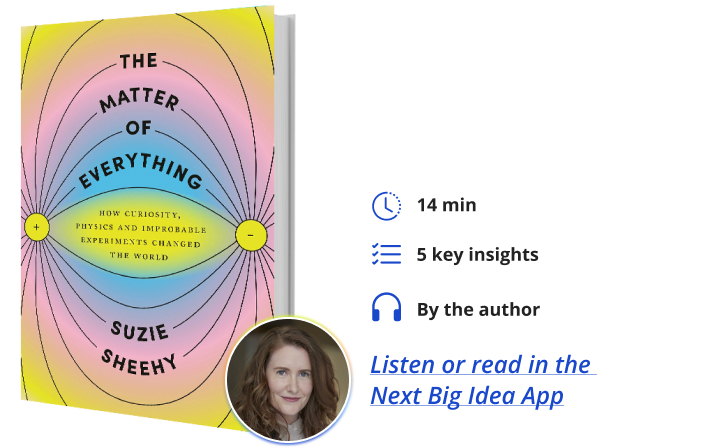
1. How we know something is just as important as what we know.
Experiments are massively under-celebrated when we learn about physics in popular culture. Most—though not all—physicists come in one of two flavors: the theorist or the experimentalist. Theorists—like Albert Einstein, Werner Heisenberg, or Max Planck—tend to work with mathematics, developing new conceptual ideas of how the world works and working through their calculations to predict or match measurements. Experimentalists have a more nuanced job: while they understand the mathematics, they have to be willing to go beyond it. Experiments rely on practical skills, physical insight, and a whole lot of persistence. They are important because we can’t know anything at all about the real world without experiments.
There are two ways that experiments can lead us to new discoveries. The first, which you might be familiar with, is the idea that a theory or hypothesis—often put forward by a theoretical physicist—predicts something, and we go out and build an experiment to test it.
This is what JJ Thomson did in 1897 when he was trying to figure out if the glowing rays he and others had seen in glass tubes, called “cathode ray tubes,” were made of particles or a form of light. His theory was that they were particles, so he designed experiments to figure out if the rays carried electric charge, and if they had a mass. With a set of clever experiments, he overcame experimental problems that had plagued previous researchers, and established that cathode rays were in fact tiny, charged particles, the first subatomic particles, which we now call electrons.
A much more recent example is the prediction of the Higgs boson, first predicted in 1964 by three theorists, Brout, Englert, and Higgs. It took decades, thousands of people, and billions of dollars to build the experiment—the Large Hadron Collider—which was able to discover the Higgs boson in 2012. The three people who predicted it almost immediately got a Nobel Prize. But the experimenters? Well, I bet you can’t name even one of them.
“Experiments rely on practical skills, physical insight, and a whole lot of persistence.”
The second way that experiments can give us new knowledge, is the more serendipitous route: when experiments produce results that aren’t predicted in advance. Like in 1896 when Wilhelm Roentgen working in Germany saw a glowing screen across his lab out of the corner of his eye and decided to investigate, leading to the discovery of X-rays—he even took the first X-ray image of his wife’s hand.
Doing experiments requires the ability to ask good questions, and the persistence to see them through.
2. The results of curiosity driven research grow in usefulness over time.
New discoveries make new imaginings possible and can lead to new creative and innovative ventures. Curiosity-driven research tends to lead to fundamentally new ideas that compound in utility over time.
Within a couple of years of discovering the electron, Thomson had explained how electrons flow out of electrodes and into glass vacuum tubes, which turned out to be exactly the knowledge that engineers needed to create vacuum valves, amplifiers, and other devices. This gave birth to the entire electronics industry, radio, telecommunications, and computers. Without these electronic valves, rock ’n’ roll would never have happened because we wouldn’t have had beautiful tube amplifiers that created that iconic sound.
And X-rays? Just two weeks later, artists were exploring a new medium taking X-ray photographs of an iguana, and doctors started using X-rays to see inside patients without a single incision in the skin. In time, X-rays became even more powerful as they were combined with other ideas. From astrophysics to hospitals to border security and industry, X-rays found more and more uses in our society.
Sometimes the biggest breakthroughs come from unexpected directions, like Charles Wilson’s cloud chamber. Initially designed to study meteorology, it became the first particle detector, letting us see the otherwise invisible passage of tiny particles. Physicists adventured with these chambers up mountains, enabling discoveries of new particles like positrons and muons, transforming forever our understanding of what matter is and how our universe works. Today, we use muons to see inside volcanoes and pyramids, and use positrons—a type of antimatter—in PET scanners in hospitals.
This path to new ideas is often nonlinear, serendipitous. What is important here is not that we know in advance where our research will lead—otherwise, it wouldn’t be research. What is of value here is simply our desire to know, and our willingness to do the work to find out.
3. Science might be objective, but scientists are not.
At the turn of the 20th century, many physicists thought their work was “done.” In 1894, Albert Michelson said, “It seems probable that most of the grand underlying principles have been firmly established,” and in 1901 Lord Kelvin proclaimed, “The future truths of physical sciences are to be looked for in the sixth place of decimals.” They couldn’t have been more wrong. What came after was a complete revolution in our understanding, from the unstable nature and inherent emptiness of atoms to quantum mechanics. Yet it’s hard to predict the future because our imaginations are limited by our current theoretical ideas and technological capability.
“We are limited in our capacity to imagine what might be possible, or how the world might really operate.”
So we shouldn’t forget that physicists—contrary to many pop culture representations—are only people. We are limited in our capacity to imagine what might be possible, or how the world might really operate. Perhaps this is why our discoveries sometimes have to happen serendipitously. Physicists are not immune to groupthink, egos, bias, or any other vice! We’ve had to work really hard to overcome these biases in our methods.
Even when we do make amazing discoveries, some ideas in physics are so counterintuitive that they take a long time to be accepted. Experimenter Robert Millikan was not alone in dismissing early ideas of quantum mechanics, which he called a “reckless” hypothesis. He spent 12 years in the lab doing experiments to disprove Einstein’s theories on the “photoelectric effect,” all the while gathering the most precise data yet that showed Einstein was right.
The way through this very human vulnerability is both the simplest and hardest aspect of doing research. We need to cultivate a culture of curiosity and stay open to the idea that we might be wrong.
4. Who gets to be a physicist?
We need the best and brightest minds to work on the most difficult problems in science. Curiosity is a human trait. It isn’t racist or sexist. But sadly it turns out that for most of the history of science, we have been limiting who can contribute. And it is our loss.
When I learned physics, and in my research for this book, I couldn’t help but notice that the “great white man narrative” of science was strong in the history of my field. Yet there were women working in the field. In my research I found the stories of amazing physicists like Harriet Brooks, whose experiments in the early 1900s helped us understand that radioactive elements could change or “transmute” into one another. Or Marietta Blau, whose unique background in both photography and physics inspired her to make a type of particle detector—known as a nuclear emulsion—that led to countless discoveries and won a number of other people Nobel Prizes, but not Blau, despite her being nominated multiple times. Or Indian physicist Bibha Chowdhuri, whose first-authored Nature paper on her discovery of two different new particles wasn’t enough to clinch her the Nobel, which went to a man a few years later for the same discovery, albeit with his superior equipment.
These stories are not isolated. Women have always been interested in physics, and have always made amazing contributions, but they have been discouraged, shut out, and had those contributions overlooked or even attributed to their male colleagues. Our society tells women that they don’t look like physicists because we don’t have stories of the women physicists who went before. This isn’t a few isolated instances, it has a name: the Matilda Effect, named by science historian Margaret Rossiter after Matilda Gage, a suffragist who first recognized that women’s contributions are often overlooked or neglected.
5. Collaboration is the human force of nature.
At first, chance led to physicists working across disciplinary boundaries: one physicist had a brother who was a medical doctor, another found a collaborator at their new university. Later, more intentional collaborations emerged, like the physicists who used high power radar technology to shrink down particle accelerators, so that now we find them in hospitals treating cancer around the world.
“They also realized that a common project to pursue peaceful scientific goals could have the effect of bridging the divide between countries that had formerly been at war.”
But even more powerful was the way physicists learned to collaborate across international borders. After WWII, many European countries were financially decimated, and the scientists living there realized they could not compete to answer big scientific questions with the small resources available. They also realized that a common project to pursue peaceful scientific goals could have the effect of bridging the divide between countries that had formerly been at war. In 1954 the convention was signed ratifying CERN: The European Laboratory for Particle Physics, now home of the Large Hadron Collider, birthplace of the World Wide Web and many other inventions, a hotbed of discovery and curiosity. Nowadays, CERN even teaches the UN how to collaborate.
The problems facing us today are too large for a few individuals, companies, or even a single nation to solve. And I’m not just talking about physics. To my surprise, I found that when I had traced the incredible journey of 120 years of experiments in physics, a new perspective emerged. In trying to understand the fundamental nature of matter and the forces that have shaped our universe, perhaps the greatest thing that physicists have taught us is about a much more human force: the power of collaboration, and of learning to work together toward something greater than ourselves.
To listen to the audio version read by author Suzie Sheehy, download the Next Big Idea App today:
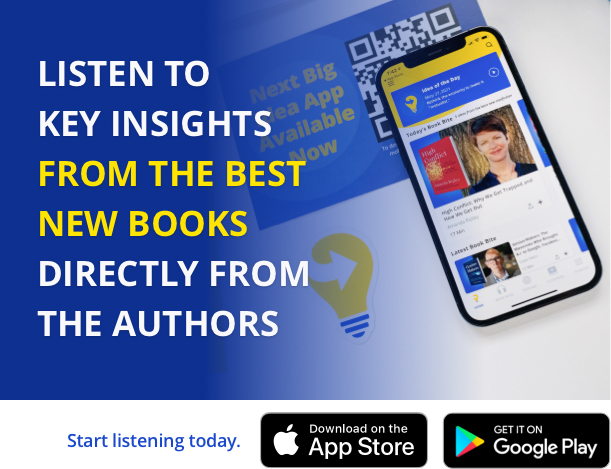